ABSTRACT
The Internet of Underwater Things (IoUT) is a novel class of Internet of Things (IoT), and is defined as the network of smart interconnected underwater objects. IoUT is expected to enable various practical applications, such as environmental monitoring, underwater exploration, and disaster prevention. With these applications, IoUT is regarded as one of the potential technologies toward developing smart cities. To support the concept of IoUT, Underwater Wireless Sensor Networks (UWSNs) have emerged as a promising network system. UWSNs are different from the traditional Territorial Wireless Sensor Networks (TWSNs), and have several unique properties, such as long propagation delay, narrow bandwidth, and low reliability.
These unique properties would be great challenges for IoUT. In this paper, we provide a comprehensive study of IoUT, and the main contributions of this paper are threefold: (1) we introduce and classify the practical underwater applications that can highlight the importance of IoUT; (2) we point out the differences between UWSNs and traditional TWSNs, and these differences are the main challenges for IoUT; and (3) we investigate and evaluate the channel models, which are the technical core for designing reliable communication protocols on IoUT.
APPLICATIONS
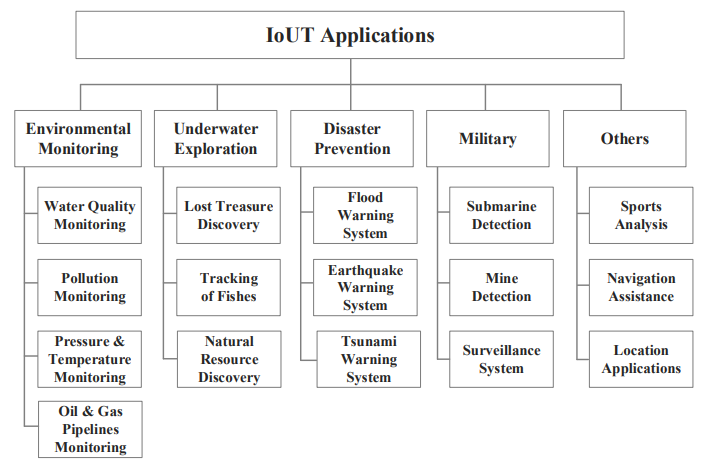
Table 1. The differences between TWSNs and UWSNs
In the last decade, researchers have presented numerous practical and potential IoUT applications. We classify the applications into five types: (1) environmental monitoring; (2) underwater exploration; (3) disaster prevention; (4) military; and (5) others (Figure 2).
CHALLENGES
In summary, the differences (between TWSNs and UWSNs) are the main challenges for IoUT. On the one hand, the differences cause many challenging issues for IoUT; on the other hand, the challenging issues attract research on IoUT. In recent years, researchers have discussed and tackled some of the challenging issues.
Although extensive research has been done on the issues of the propagation delay, bandwidth utilization, and energy efficiency, few studies have focused on the more fundamental issue of link reliability. As we can imagine, low reliability would lead to frequent data retransmission; then, frequent retransmission will eventually result in longer delay, higher bandwidth consumption, and higher energy consumption.
CHANNEL MODELS
To calculate the reliability of links on IoUT, we investigate the channel models for underwater environments. The aim of the models is to calculate the successful delivery ratio of each link over UWSNs. The successful delivery ratio (between a pair of sensor nodes) is equivalent to the reliability (of a link), and is one of the most advantageous information for designing reliable communication protocols on IoUT.
The underwater channel models consist of several elements. We divide the complicated channel models into two main parts. In the first part, we investigate the relationship between the transmitter power and signal-to-noise ratio (SNR); then, in the second part, we further investigate the relationship between the SNR and successful delivery ratio. To sum up, the channel models can provide researchers with a systematic way to calculate the reliability (i.e., successful delivery ratio) of each link over IoUT.
RESULTS
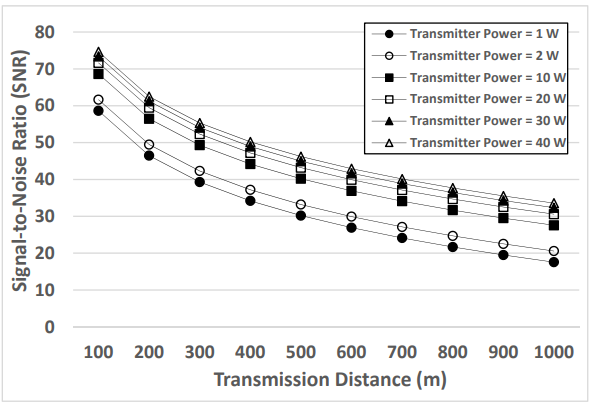
Figure 3. Simulation results of the Signal-to-Noise Ratio (SNR) when the channel models are applied to different transmitter powers (1–40W) and distances (100–1000m)
Figure 3 shows the simulation results of the SNR when the transmitter powers are set as 1W, 2W, 10W, 20W, 30W, and 40W. This figure plots the SNR as a function of the transmission distance. The distances are from 100m to 1000m.
First, when the transmission distance increases, the SNR decreases. This is because the “transmission loss” is one of the main elements of the SNR. When the distance increases, the transmission loss would be accumulated; subsequently, the accumulated transmission loss makes a negative impact on the SNR. Therefore, the SNR is inversely proportional to the transmission distance between the underwater sensor nodes.
Second, when the transmitter power is higher, the SNR is higher. Specifically, when the transmission distance is the same, the transmitter powers from low SNR to high SNR are: 1W, 2W, 10W, 20W, 30W, and then 40W. This is because one of the main elements of the SNR is the “source level”; when the transmitter power is higher, the source level is higher.
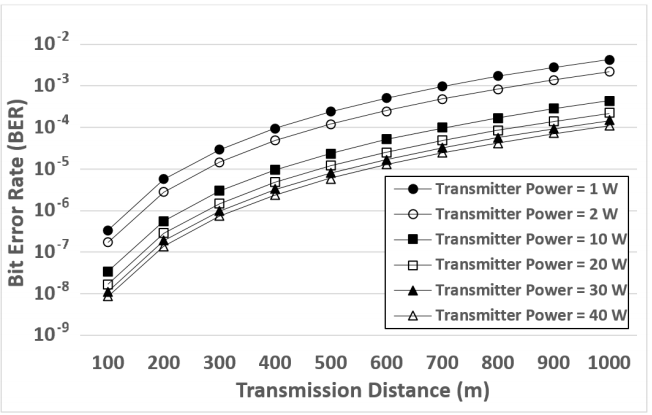
Figure 4. Simulation results of the Bit Error Rate (BER) when the channel models are applied to different transmitter powers (1–40W) and distances (100–1000m)
Figure 4 shows the simulation results of the BER. This figure plots the BER as a function of the transmission distance. The transmission distances are from 100m to 1000m, and the transmitter powers are set as 1W, 2W, 10W, 20W, 30W, and 40W.
DISCUSSION
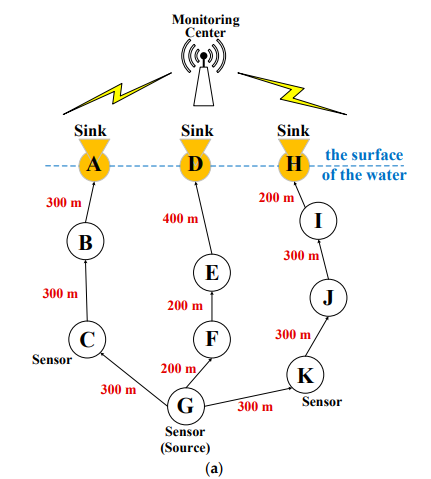
Figure 6. Example of the routing paths in UWSNs with: (a) the information of link distance
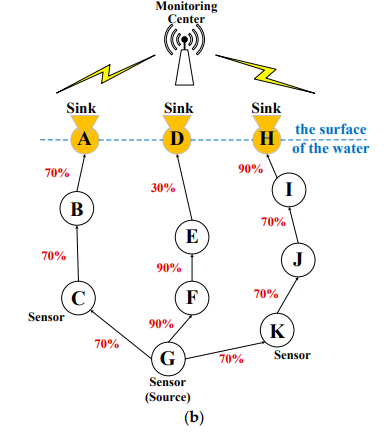
Figure 6. Example of the routing paths in UWSNs with: (b) the information of link reliability
Figure 6 shows a typical example. In Figure 6a, the shortest path from the source sensor node (i.e., node G) to the sink (i.e., any sink on the surface of the water) is: node G, node F, node E, and then sink D. The distance of the shortest path is: 200m + 200m + 400m = 800m. However, as shown in Figure 6b, the most reliable path in the same UWSN is a different path: node G, node C, node B, and then sink A.
This is because the reliability of the most reliable path is: 70% × 70% × 70% = 34.3%. This is one simple example to point out the importance of the reliability between a pair of sensor nodes in UWSNs. Without the information of the link reliability, it would be very difficult for a routing protocol to select the most reliable path.
RELATED WORK
In this section, we introduce three state-of-the-art IoUT-related works. These works can provide more information of IoUT, and offer the potential research directions in the near feature.
In, the research effort has focused on the MAC-layer packet scheduling for IoUT. Considering the design challenges of MAC protocols, the authors presented an Energy-Aware scheduling with Spatial-Temporal reuse (EAST) protocol for IoUT. The authors mentioned the design challenges of the IoUT MAC protocols, including: (1) sensor network lifetime; (2) acoustic channel utilization; and (3) packet loss problems. EAST utilizes the information of data buffer and traffic load to switch the energy modes (i.e., the listen and sleep mode) of underwater sensor nodes, and thereby achieves both high energy efficiency and high channel utilization.
In, the authors concentrated on the network-layer routing protocol for IoUT. Considering the IoUT challenges of data transmission and of energy efficiency, the authors proposed an Enhanced Channel-Aware Routing Protocol (E-CARP) for IoUT. E-CARP exploits the greedy strategy to deliver packets hop-by-hop. Specifically, E-CARP simplifies the relay-node selection method and reduces control packets, and thereby reduces the communication cost and energy consumption. As a result, E-CARP can reserve energy and lengthen the lifetime of the underwater sensor system for IoUT.
CONCLUSIONS
In this paper, we study a new class of IoT, called IoUT, i.e., the Internet of Underwater Things. This paper provides useful information about the IoUT: (1) applications; (2) challenges; and (3) channel models. The simulation results have confirmed that our channel models are practical and applicable to different transmitter powers (i.e., 1–40W) and to different transmission distances (i.e., 100–1000m). Specifically, when the transmitter power is higher, the corresponding SNR is higher, the BER is lower, and thereby the successful delivery ratio is higher.
Furthermore, when the transmission distance increases, the corresponding SNR decreases, and the BER increases, the delivery ratio decreases. The channel models are reasonable, and are expected to help researchers investigate the communication protocols on IoUT. In the future, we will also apply the channel models to further investigate the design of different IoUT communication protocols, such as the MAC protocols and routing protocols.
Source: National Taiwan Ocean University
Authors: Chien-Chi Kao | Yi-Shan Lin | Geng-De Wu | Chun-Ju Huang
>> Top Sensor based IoT Projects for ECE/EEE Students
>> 50+ IoT based Wireless/GSM Projects for Engineering Students
>> 200+ IoT Led Engineering Projects for Students
>> IoT Environmental Monitoring System for Smart Cities Projects